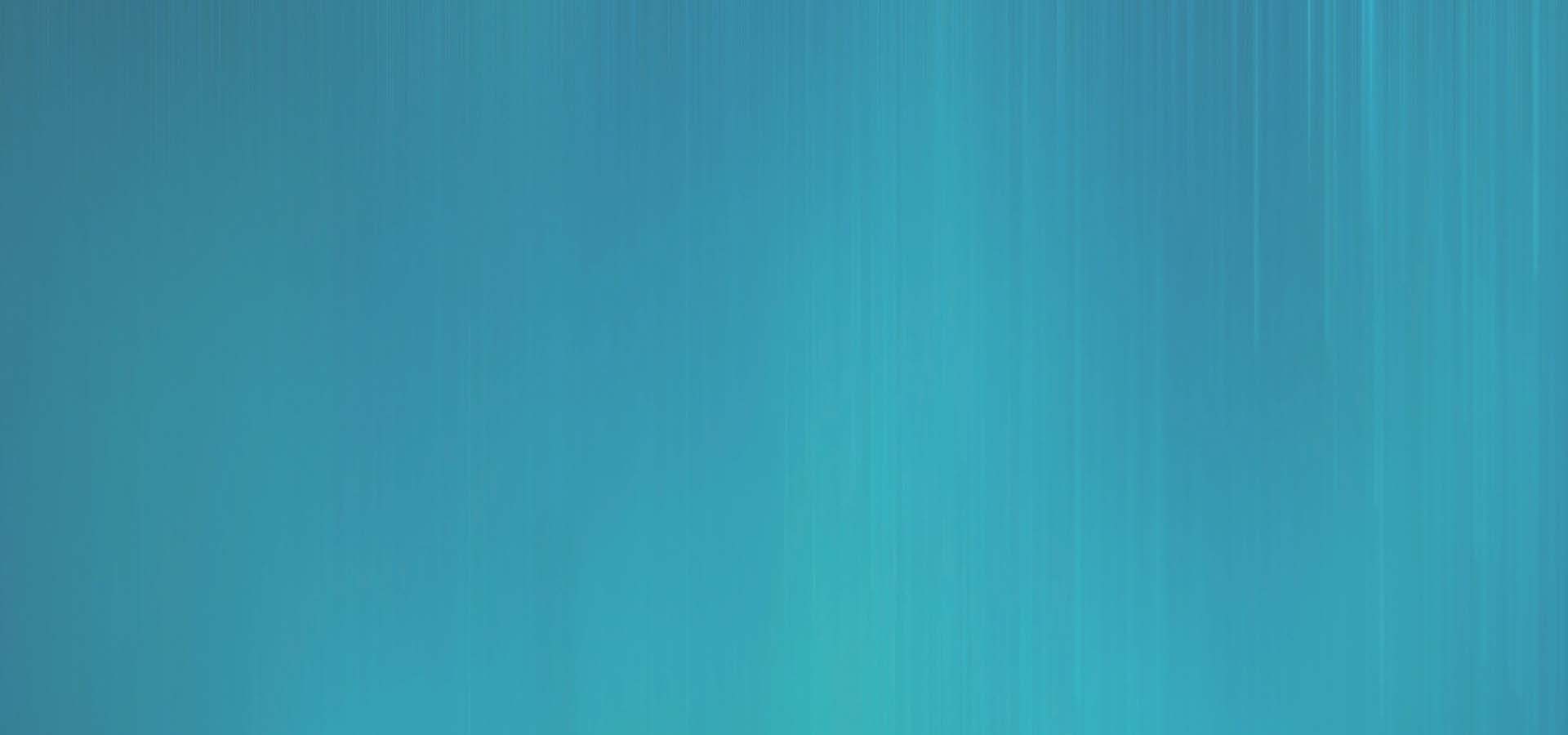
Optical beam-deflection atomic force microscopy system at low temperature
Research Project | 2 Project Members
This project aims to develop an advanced optical beam-deflection atomic force microscope (AFM) integrated in a low-temperature cryostat and an ultra-high vacuum system. The system will enable precise study of individual molecules and complex 2D molecular systems. The cryostat, optimized for low helium consumption and long hold times, will support extended experiments, while the vacuum environment will allow in-situ preparation of samples through methods like thermal evaporation and electrospray deposition. The AFM, built at the University of Basel, will include a preamplifier to extend bandwidth and enable faster data acquisition, supporting advanced AFM modes such as multimode AFM. The system will also feature precise positioning of the AFM probe on 2D materials and quantum dot devices, using optical microscopy and large-area scanning. Controlled electrostatic potentials and back-gate voltages will allow for quantum dot confinement and charge density manipulation, facilitating experiments like 2D or 3D force spectroscopy. Molecules will be studied at submolecular resolution, allowing for manipulation and measurement of lateral forces. Examples include investigating molecular knots and nanographene, with a focus on processes like dehydrogenation. Frictional forces on different substrates will also be explored. Finally, high-resolution AFM and Kelvin probe force microscopy (KPFM) will be used to map charge distributions in electron donor-acceptor systems, including those in excited states, offering new insights into molecular interactions and dynamics.