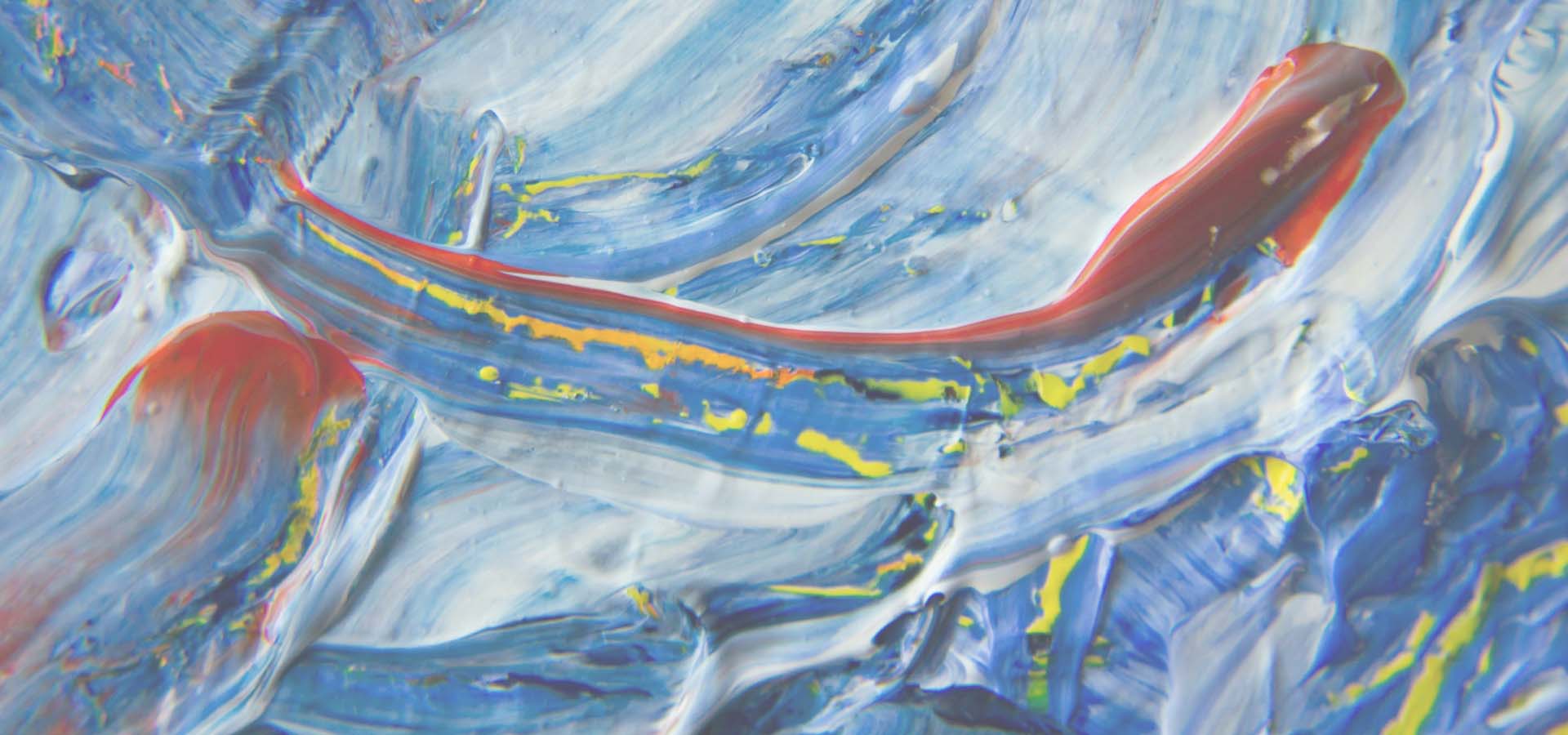
Nanopatterning and precision-polymer doping: towards single dopant quantum devices
Research Project | 1 Project Members
Our objective is to develop and experimentally validate a new combination of nanopatterning and deterministic doping of phosphorous (P) in silicon (Si) that is required for the manufacturing of quantum devices. Our approach is based on thermal scanning probe lithography (t-SPL), directed self-assembly of block-copolymers (DSA-BCP), monolayer/polymeric-precision doping (MLD/PPD) and rapid thermal annealing (RTA) to create micro and nanopatterns of P-doped areas in Si, down to single atom doping control. The project will first fabricate test patterns (1 um – 20 nm) having variable geometries and dopant concentration inside the Si crystal, e.g. 1D, 2D arrays, with multiple dopant patterns, that allow us to assess the local dopant profile, and to optimize the process by varying systematically fabrication parameters. To ensure single P dopant control, molecule-receiving holes of around 5 nm are needed, which will be achieved using t-SPL and advanced dry etching to create a 20 nm guide pattern, which will subsequently be reduced by DSA of BCP. Inside these holes we will perform P doping by MLD/PPD using molecules of various kinds and sizes with P moieties. The doping patterns will be characterized by advanced surface analysis techniques, e.g. by Kelvin Probe Force Microscopy (KPFM). Results from the KPFM will be used in an iterative way to optimize design and process flow and to achieve single dopant control in a deterministic way. In a second phase, besides continuing to optimize the doping process, we will begin exploring the use of the single doping technology towards concrete quantum devices. We aim to demonstrate that the technology can be incorporated into the standard process flow for donor-based semiconductor devices as for example, single atom point contact transistors, artificial lattices in semiconductors and deterministic doping for semiconducting qubits. Each PhD topic will be aligned to one of the configurations according to status of the advances we can make. The proposed work requires expertise in nanofabrication, self-assembly and ultra-high-resolution surface and device characterization, The project team with members from EPFL, UniBasel and CNM-Barcelona has been assembled to gather the most advanced skill set in the respective project parts present. Our research project will train four new PhD students in a timely topic related to nanomanufacturing and quantum technology.