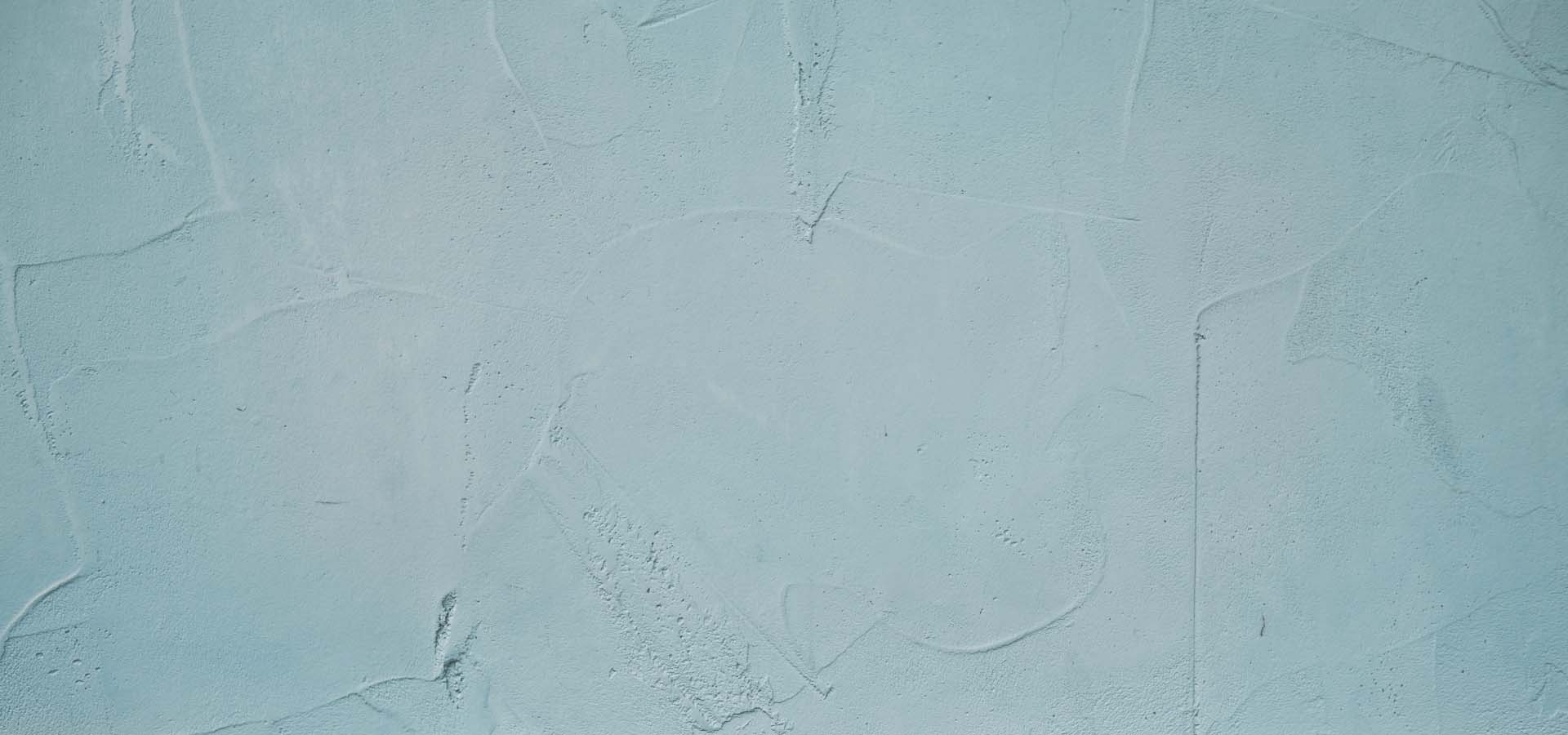
Catch Bond Mediated Peristaltic Hydrogels
Research Project | 2 Project Members
From the beating of our hearts, to the wave-like (peristaltic) motion of our intestines, the human body is a hive of activity. Dynamic processes are not only essential to our health but also to the differentiation, growth and regeneration of motile tissues, in particular, the intestines. Despite strong interest in the regeneration of intestinal tissue and gaining a clearer insight into the role of peristalsis in intestinal function, matrices used for the culture of intestinal organoids (IOs) and tissues are static. This limits their quality and viability and poses an obstacle to the accuracy of IO models and regenerative medicine. However, hydrogel matrices that exhibit the peristaltic motion required by intestinal tissues, specifically motion that is responsive to environmental cues, do not currently exist. Hydrogels cross-linked by responsive protein receptor-ligand (RL) complexes have the potential to harness the unique properties of their protein cross-links on the macroscale, including their response to mechanical and chemical stimulation. The main objective of the proposed research is to incorporate mechanoresponsive protein complexes into a hydrogel matrix, quantify their ability to alter the viscoelastic properties of the hydrogel as a function of environmental stimuli and assess the impact of this dynamic behaviour on cultured IOs. Combining microscale (AFM imaging and microrheology) and macroscale (rheology and mechanical testing) analytical techniques with biochemical characterisation (fluorescence microscopy and flow cytometry) and prior knowledge of biopolymer chemistry, I will rationally design biopolymer scaffolds, in which mechanically active proteins are embedded, and examine their responsive behaviour alongside their ability to support IO growth. By examining both the influence of the IOs on the hydrogel and that of the hydrogels on the IOs, knowledge of dynamic behaviour will be obtained in a cyclic manner that will permit tuning of the mechanical and chemical responsivity of the matrices to optimise dynamic behaviour and the impact on the cultured IOs. A rationally designed dynamic hydrogel that can adjust its viscoelastic behaviour in a periodic manner in response to local chemical and mechanical cues and support the growth of accurate and viable IOs will be the successful conclusion of this project. Insights gained in this study will lay the foundations for further exploration of responsive protein complexes in the design of dynamic cell culture matrices and their applicability to other organoid and tissue types, ultimately leading to significant leaps in organoid technology and regenerative medicine.